Synthesis and application of mixed spinel Mn0.4Cd0.6Cr2S2Se2: Structural, magnetic, and electrochemical sensing properties
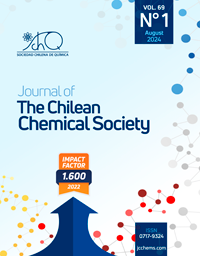
- Chalcospinel,
- electrochemical sensor,
- ferromagnetism,
- single crystal
Copyright (c) 2024 SChQ

This work is licensed under a Creative Commons Attribution-NonCommercial-ShareAlike 4.0 International License.
Abstract
Herein we report the solid synthesis, structural characterization, magnetic behavior, and electrochemical sensing properties of Mn0.4Cd0.6Cr2S2Se2. Single‐crystal X‐ray diffraction analysis showed that Mn0.4Cd0.6Cr2S2Se2 crystallizes in a spinel‐type structure. Powder X-ray diffraction patterns and Rietveld refinement data revealed that this selenide phase is consisted of cubic Fdm space group. Magnetic field cooling (MFC) measurements indicated an enhancement in ferromagnetic interactions relative to the ferrimagnetic compound Mn0.4Cd0.6Cr₂S₄, which can be attributed to the substitution of sulphur by selenium. The electrochemical response of modified glassy carbon electrodes with Mn0.4Cd0.6Cr2S2Se2 was increased, the peak current is increased 4-fold, from 20.15 µA to 83.52 for GC, and GC-Mn0.4Cd0.6Cr2S2Se2 respectively by differential pulse voltammetry, and thus it could be used to design an electrochemical sensor to quantify nitrocompounds, considered pollutants and toxic agents for humans, plants, and animals.
References
- (1) Ganesh, K. S.; Gutturu, R. R.; Kang, C. S.; Joo, S. W. Characteristics of Nanoparticles of Mixed Cu–Zn–Mg Spinel Ferrites: A Study of Structural and Electrochemical Attributes. Ceram Int 2024, 50 (4), 6268–6277. https://doi.org/10.1016/j.ceramint.2023.11.352.
- (2) Qin, H.; He, Y.; Xu, P.; Huang, D.; Wang, Z.; Wang, H.; Wang, Z.; Zhao, Y.; Tian, Q.; Wang, C. Spinel Ferrites (MFe2O4): Synthesis, Improvement and Catalytic Application in Environment and Energy Field. Advances in Colloid and Interface Science. Elsevier B.V. August 1, 2021, pp 1–22. https://doi.org/10.1016/j.cis.2021.102486.
- (3) Nguyen, V. C.; Kim, H. Spinel Nanoparticles ZnCo2O4 as High Performance Electrocatalyst for Electrochemical Sensing Antibiotic Chloramphenicol. Journal of Electrochemical Science and Technology 2024, 15 (1), 152–160. https://doi.org/10.33961/jecst.2023.00486.
- (4) Gonçalves, J. M.; de Faria, L. V.; Nascimento, A. B.; Germscheidt, R. L.; Patra, S.; Hernández-Saravia, L. P.; Bonacin, J. A.; Munoz, R. A. A.; Angnes, L. Sensing Performances of Spinel Ferrites MFe2O4 (M = Mg, Ni, Co, Mn, Cu and Zn) Based Electrochemical Sensors: A Review. Analytica Chimica Acta. Elsevier B.V. November 15, 2022. https://doi.org/10.1016/j.aca.2022.340362.
- (5) Palpandi, K.; Bhuvaneswari, C.; Babu, S. G.; Raman, N. Rational Design of Ruddlesden-Popper Phase Mn2SnO4 for Ultra-Sensitive and Highly Selective Detection of Chloramphenicol in Real-Life Samples. New Journal of Chemistry 2022. https://doi.org/10.1039/d2nj00813k.
- (6) Ma, X.; Xiao, M.; Yang, X.; Guo, Y.; Yu, X.; Ge, M. Tuning Benzene Activity of Cobalt-Based Spinel Catalysts with Cation Substitution. Catal Today 2024, 436. https://doi.org/10.1016/j.cattod.2024.114770.
- (7) Khan, M. Z. H.; Zhu, J.; Liu, X. Reduced Graphene Oxide-Conjugated Urchin-like NiCo2O4 Nanostructures for Individual Detection of o-Nitro and p-Amino Phenol. ACS Omega 2019, 4 (7), 11433–11439. https://doi.org/10.1021/acsomega.9b00804.
- (8) Ramu, A. G.; Salla, S.; Gopi, S.; Silambarasan, P.; Yang, D. J.; Song, M. J.; Ali, H. M.; Salem, M. Z. M.; Choi, D. Surface-Tuned Hierarchical ɤ-Fe2O3–N-RGO Nanohydrogel for Efficient Catalytic Removal and Electrochemical Sensing of Toxic Nitro Compounds. Chemosphere 2021, 268. https://doi.org/10.1016/j.chemosphere.2020.128853.
- (9) Zhang, J.; Cui, S.; Ding, Y.; Yang, X.; Guo, K.; Zhao, J. T. Two-Dimensional Mesoporous ZnCo2O4 Nanosheets as a Novel Electrocatalyst for Detection of o-Nitrophenol and p-Nitrophenol. Biosens Bioelectron 2018, 112, 177–185. https://doi.org/10.1016/j.bios.2018.03.021.
- (10) Laganovsky, A. V.; Kormosh, Z. O.; Sachanyuk, V. P.; Parasyuk, O. V. Quaternary Thiospinel CuCrTiS4 as an Electroactive Material for Copper(II)-Sensitive and Selective Electrode. Materials Science and Engineering C 2008, 28 (7), 1112–1116. https://doi.org/10.1016/j.msec.2007.05.002.
- (11) Balamurugan, K.; Rajakumaran, R.; Chen, S. M.; Karthik, R.; Shim, J. J.; Shafi, P. M. Massive Engineering of Spinel Cobalt Tin Oxide/Tin Oxide-Based Electrocatalyst for the Selective Voltammetric Determination of Antibiotic Drug Furaltadone in Water Samples. J Alloys Compd 2021, 882. https://doi.org/10.1016/j.jallcom.2021.160750.
- (12) Chen, T. W.; Tamilalagan, E.; Chen, S. M.; Akilarasan, M.; Maheshwaran, S.; Liu, X. An Ultra-Sensitive Electrochemical Sensor for the Detection of Carcinogen Oxidative Stress 4nitroquinoline N-Oxide in Biologic Matrices Based on Hierarchical Spinel Structured NiCo2O4 and NiCo2S4; a Comparative Study. Int J Mol Sci 2020, 21 (9). https://doi.org/10.3390/ijms21093273.
- (13) Palpandi, K.; Raman, N. Electrochemical Detection of 2-Nitroaniline at a Novel Sphere-like Co2SnO4 Modified Glassy Carbon Electrode. New Journal of Chemistry 2020, 44 (20), 8454–8462. https://doi.org/10.1039/d0nj01098g.
- (14) Koventhan, C.; Vinothkumar, V.; Chen, S. M. Development of an Electrochemical Sensor Based on a Cobalt Oxide/Tin Oxide Composite for Determination of Antibiotic Drug Ornidazole. New Journal of Chemistry 2021, 45 (28), 12593–12605. https://doi.org/10.1039/d1nj01345a.
- (15) Zhao, Q.; Yan, Z.; Chen, C.; Chen, J. Spinels: Controlled Preparation, Oxygen Reduction/Evolution Reaction Application, and Beyond. Chemical Reviews. American Chemical Society August 9, 2017, pp 10121–10211. https://doi.org/10.1021/acs.chemrev.7b00051.
- (16) Shen, J.; Dong, P.; Baines, R.; Xu, X.; Zhang, Z.; Ajayan, P. M.; Ye, M. Controlled Synthesis and Comparison of NiCo2S4/Graphene/2D TMD Ternary Nanocomposites for High-Performance Supercapacitors. Chemical Communications 2016, 52 (59), 9251–9254. https://doi.org/10.1039/c6cc03699f.
- (17) Bulakhe, R. N.; Ryu, C.; Gunjakar, J. L.; In, J. Bin. Chemical Route to the Synthesis of Novel Ternary CuCr2S4 Cathodes for Asymmetric Supercapacitors. J Energy Storage 2022, 56. https://doi.org/10.1016/j.est.2022.106175.
- (18) Yu, X. Y.; (David) Lou, X. W. Mixed Metal Sulfides for Electrochemical Energy Storage and Conversion. Advanced Energy Materials. Wiley-VCH Verlag January 25, 2018. https://doi.org/10.1002/aenm.201701592.
- (19) Yu, Z.; Tetard, L.; Zhai, L.; Thomas, J. Supercapacitor Electrode Materials: Nanostructures from 0 to 3 Dimensions. Energy and Environmental Science. Royal Society of Chemistry March 1, 2015, pp 702–730. https://doi.org/10.1039/c4ee03229b.
- (20) Abbasi, J.; Regmi, S.; Gupta, A. Ferromagnetic Cd(1-x)CuxCr2S4 Thin Films: Synthesis, Characterization, and First-Principles Calculations. J Magn Magn Mater 2022, 562. https://doi.org/10.1016/j.jmmm.2022.169771.
- (21) Tsurkan, V.; Krug von Nidda, H. A.; Deisenhofer, J.; Lunkenheimer, P.; Loidl, A. On the Complexity of Spinels: Magnetic, Electronic, and Polar Ground States. Physics Reports. Elsevier B.V. September 3, 2021, pp 1–86. https://doi.org/10.1016/j.physrep.2021.04.002.
- (22) Ohgushi, K.; Okimoto, Y.; Ogasawara, T.; Miyasaka, S.; Tokura, Y. Magnetic, Optical, and Magnetooptical Properties of Spinel-Type ACr2X4 (A = Mn, Fe, Co, Cu, Zn, Cd; X = O, S, Se). J Physical Soc Japan 2008, 77 (3). https://doi.org/10.1143/JPSJ.77.034713.
- (23) Valencia-gálvez, P.; Peña, O.; Moris, S.; Barahona, P. RAMAN CHARACTERIZATION OF CuCr2-xSnxS4 SPINELS. J. Chil. Chem. Soc 2019, 64 (1), 4285–4289. https://doi.org/10.4067/s0717-97072019000104285.
- (24) Ozel, F.; Kılıc, H. S.; Coskun, H.; Deveci, I.; Sarılmaz, A.; Balıkcıoglu, A.; Gundogdu, Y.; Aljabour, A.; Ozen, A.; Gezgin, S. Y.; Houimi, A.; Yar, A.; Kus, M.; Ersoz, M. A General Review on the Thiospinels and Their Energy Applications. Mater Today Energy 2021, 21, 100822. https://doi.org/https://doi.org/10.1016/j.mtener.2021.100822.
- (25) Barahona, P.; Galdámez, A.; López-Vergara, F.; Manríquez, V.; Peña, O. Crystal Structure and Magnetic Properties of Titanium-Based CuTi2-xMxS4 and CuCr2-xTixSe4 Chalcospinels. J Solid State Chem 2014, 212, 114–120. https://doi.org/10.1016/j.jssc.2014.01.017.
- (26) Moris, S.; Valencia-Gálvez, P.; Mejía-López, J.; Peña, O.; Barahona, P.; Galdámez, A. (Cu)Tet(Cr2- xSnx)OctS4- ySey Spinels: Crystal Structure, Density Functional Theory Calculations, and Magnetic Behavior. Inorg Chem 2019, 58 (20), 13945–13952. https://doi.org/10.1021/acs.inorgchem.9b01853.
- (27) Pinto, C.; Galdámez, A.; Barahona, P.; Moris, S.; Peña, O. Crystal Structure, Raman Scattering and Magnetic Properties of CuCr2−xZrxSe4 and CuCr2−xSnxSe4 Selenospinels. J Magn Magn Mater 2018, 456, 160–166. https://doi.org/10.1016/j.jmmm.2018.02.023.
- (28) Moris, S.; Barahona, P.; Galdámez, A. Crystal Structure of (Cu0.51In0.49 )Tet[Cr1.74In0.26]OctSe4 Selenospinel, Cu0.51In0.75Cr1.74Se4. Zeitschrift für Kristallographie-New Crystal structure 2019, 234 (3), 421–422. https://doi.org/10.1515/ncrs-2018-0426.
- (29) Valencia‐gálvez, P.; Aravena, D.; Barahona, P.; Moris, S.; Galdámez, A. Effects of Tin and Sulfur Chemical Substitution on the Structural and Electrical Properties of CuCr2Se4 Selenospinel. Applied Sciences (Switzerland) 2022, 12 (3). https://doi.org/10.3390/app12031586.
- (30) Barahona, P.; Galdamez, A.; Manríquez, V.; Cruzat, C.; Raison, C.; Le Coz, S.; Peña, O.; Moure, C. Effect of Cation Substitution on Magnetic Properties in Mn1-XA’xCr2S4 (A’ = Cd, Zn) Thiospinel Series. Advances in Applied Ceramics 2010, 109 (7), 431–435. https://doi.org/10.1179/174367609X414125.
- (31) Abdel-Aziz, A. M.; Hassan, H. H.; Badr, I. H. A. Activated Glassy Carbon Electrode as an Electrochemical Sensing Platform for the Determination of 4-Nitrophenol and Dopamine in Real Samples. ACS Omega 2022, 7 (38), 34127–34135. https://doi.org/10.1021/acsomega.2c03427.
- (32) Zeng, Y.; Zhou, Y.; Zhou, T.; Shi, G. A Novel Composite of Reduced Graphene Oxide and Molecularly Imprinted Polymer for Electrochemical Sensing 4-Nitrophenol. Electrochim Acta 2014, 130, 504–511. https://doi.org/10.1016/j.electacta.2014.02.130.
- (33) Zhang, J.; Cui, S.; Ding, Y.; Yang, X.; Guo, K.; Zhao, J. T. Two-Dimensional Mesoporous ZnCo2O4 Nanosheets as a Novel Electrocatalyst for Detection of o-Nitrophenol and p-Nitrophenol. Biosens Bioelectron 2018, 112, 177–185. https://doi.org/10.1016/j.bios.2018.03.021.
- (34) Thirumalraj, B.; Rajkumar, C.; Chen, S. M.; Lin, K. Y. Determination of 4-Nitrophenol in Water by Use of a Screen-Printed Carbon Electrode Modified with Chitosan-Crafted ZnO Nanoneedles. J Colloid Interface Sci 2017, 499, 83–92. https://doi.org/10.1016/j.jcis.2017.03.088.
- (35) Balasubramanian, P.; Balamurugan, T. S. T.; Chen, S. M.; Chen, T. W. Simplistic Synthesis of Ultrafine CoMnO3 Nanosheets: An Excellent Electrocatalyst for Highly Sensitive Detection of Toxic 4-Nitrophenol in Environmental Water Samples. J Hazard Mater 2019, 361, 123–133. https://doi.org/10.1016/j.jhazmat.2018.08.070.
- (36) Peng, D.; Zhang, J.; Qin, D.; Chen, J.; Shan, D.; Lu, X. An Electrochemical Sensor Based on Polyelectrolyte-Functionalized Graphene for Detection of 4-Nitrophenol. Journal of Electroanalytical Chemistry 2014, 734, 1–6. https://doi.org/10.1016/j.jelechem.2014.09.027.
- (37) Sajjan, V. A.; Aralekallu, S.; Nemakal, M.; Palanna, M.; Keshavananda Prabhu, C. P.; Koodlur Sannegowda, L. Nanomolar Detection of 4-Nitrophenol Using Schiff-Base Phthalocyanine. Microchemical Journal 2021, 164. https://doi.org/10.1016/j.microc.2021.105980.
- (38) Laugier, J.; Bochu, B. CHECKCELL. Laboratoire des Matériaux et du Génie Physique Ecole Nationale Supérieure de Physique de Grenoble (INPG): Grenoble October 22, 2010. https://www.inpg.fr/LMGP (accessed 2024-06-05).
- (39) Rodríguez-Carvajal, J. Recent Advances in Magnetic Structure Determination by Neutron Powder Diffraction. Physica B: Physics of Condensed Matter 1993, 192 (1–2), 55–69. https://doi.org/10.1016/0921-4526(93)90108-I.
- (40) Inc., B. A. X. I. SMART, SAINTPLUS V6.02, SHELXTL V6.10 and SADABS; Bruker Analytical X-Ray Instruments Inc., Madison, Wisconsin, USA. 2015.
- (41) Sheldrick, G.M. SHELXL-97. Program for the Refinement of Crystal Structures; University of Göttingen: Stuttgart, Germany. Program for the Refinement of Crystal Structures. University of Göttingen,. 1997.
- (42) Dolomanov, O. V.; Bourhis, L. J.; Gildea, R. J.; Howard, J. A. K.; Puschmann, H. OLEX2: A Complete Structure Solution, Refinement and Analysis Program. J Appl Crystallogr 2009, 42 (2), 339–341. https://doi.org/10.1107/S0021889808042726.
- (43) Raccah, P. M.; Bouchard, R. J.; Wold, A. Crystallographic Study of Chromium Spinels. J Appl Phys 1966, 37 (3), 1436–1437. https://doi.org/10.1063/1.1708502.
- (44) Baur, W. H. The Geometry of Polyhedral Distortions. Predictive Relationships for the Phosphate Group. Acta Crystallogr B 1974, 30 (5), 1195–1215. https://doi.org/10.1107/S0567740874004560.
- (45) Wildner, M. On the Geometry of Co(II)O6 in Inorganic Compounds. Zeitschrift für Kristallographie 1992, 202, 51–70. https://doi.org/10.1524/zkri.1992.202.1-2.51.
- (46) Robinson K; Gibbs GV; Ribbe PH. Quadratic Elongation: A Quantitative Measure of Distortion in Coordination Polyhedra. Science (1979) 1971, 172 ((3983)), 567–570.
- (47) López-Vergara, F.; Galdámez, A.; Manríquez, V.; González, G. Crystal Structure and Raman Scattering Characterization of Cu2Fe1- xCoxSnS4 Chalcogenide Compounds. Solid State Sci 2015, 49, 54–60. https://doi.org/10.1016/j.solidstatesciences.2015.09.010.
- (48) Shannon, R. D. Revised Effective Ionic Radii and Systematic Studies of Interatomic Distances in Halides and Chalcogenides. Acta Crystallographica Section A 1976, 32 (5), 751–767. https://doi.org/10.1107/S0567739476001551.
- (49) Gibart, M. P.; Robbins, M.; Lambrecht, V. G. NEW FERRIMAGNETIC SPINEL COMPOSITIONS IN THE SYSTEM MCr2S4-xSex WHERE M=Fe, Co, Mn; Pergamon Press, 1973; Vol. 34.
- (50) Smilgies, D. M. Scherrer Grain-Size Analysis Adapted to Grazing-Incidence Scattering with Area Detectors. J Appl Crystallogr 2009, 42 (6), 1030–1034. https://doi.org/10.1107/S0021889809040126.